New volumes of the Encyclopædia Britannica > Volume 30, K-MOR
(479) Page 449
Download files
Complete book:
Individual page:
Thumbnail gallery: Grid view | List view
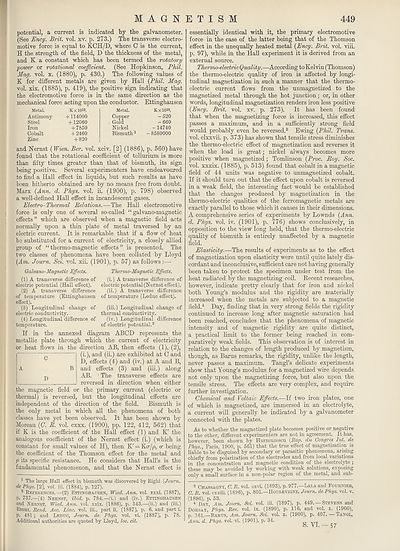
MAGNETISM
449
potential, a current is indicated by the galvanometer.
(See Ency. Brit. vol. xv. p. 273.) The transverse electro¬
motive force is equal to KCH/D, where C is the current,
H the strength of the field, D the thickness of the metal,
and K a constant which has been termed the rotatory
power or rotational coefficient. (See Hopkinson, Phil.
Mag. vol. x. (1880), p. 430.) The following values of
K for different metals are given by Hall (Phil. Mag.
vol. xix. (1885), p. 419), the positive sign indicating that
the electromotive force is in the same direction as the
mechanical force acting upon the conductor. Ettinghausen
Metal.
Antimony
Steel
Iron
Cobalt
Zinc
K x 1015.
+ 114000
+ 12060
+ 7850
+ 2460
+ 820
Metal.
Copper
Gold
Mckel
Bismuth1
K x 1015.
-520
-660
-14740
- 8580000
and Nernst (Wien. Ber. vol. xciv. [2] (1886), p. 560) have
found that the rotational coefficient of tellurium is more
than fifty times greater than that of bismuth, its sign
being positive. Several experimenters have endeavoured
to find a Hall effect in liquids, but such results as have
been hitherto obtained are by no means free from doubt.
Marx (Ann. d. Phys. vol. ii. (1900), p. 798) observed
a well-defined Hall effect in incandescent gases.
Electro-Thermal Relations.—The Hall electromotive
force is only one of several so-called “ galvano-magnetic
effects ” which are observed when a magnetic field acts
normally upon a thin plate of metal traversed by an
electric current. It is remarkable that if a flow of heat
be substituted for a current of electricity, a closely allied
group of “thermo-magnetic effects” is presented. The
two classes of phenomena have been collated by Lloyd
(Am. Journ. Sci. vol. xii. (1901), p. 57) as follows:—
Galvano-Magnetic Effects. Thermo-Magnetic Effects.
(1) A transverse difference of (i.) A transverse difference of
electric potential (Hall effect). electric potential (Nernst effect).
(2) A transverse difference (ii.) A transverse difference
of temperature (Ettinghausen of temperature (Leduc effect),
effect).
(3) Longitudinal change of (iii.) Longitudinal change of
electric conductivity. thermal conductivity.
(4) Longitudinal difference of (iv.) Longitudinal difference
temperature. of electric potential.1 2
If in the annexed diagram ABCD represents the
metallic plate through which the current of electricity
or heat flows in the direction AB, then effects (1), (2),
(i.), and (ii.) are exhibited at C and
D, effects (4) and (iv.) at A and B,
and effects (3) and (iii.) along
AB. The transverse effects are
reversed in direction when either
the magnetic field or the primary current (electric or
thermal) is reversed, but the longitudinal effects are
independent of the direction of the field. Bismuth is
the only metal in which all the phenomena of both
classes have yet been observed. It has been shown by
Moreau (G. R. vol. cxxx. (1900), pp. 122, 412, 562) that
if K is the coefficient of the Hall effect (1) and K' the
analogous coefficient of the Nernst effect (i.) (which is
constant for small values of H), then K' = Kcr/p, a being
the coefficient of the Thomson effect for the metal and
p its specific resistance. He considers that Hall’s is the
fundamental phenomenon, and that the Nernst effect is
C
A B :
D
1 The large Hall effect in bismuth was discovered by Righi {Journ.
de Phys. [2], vol. iii. (1884), p. 127).
2 References.—(2) Ettinghausen, Wied. Ann. vol. xxxi. (1887),
p. 737.—(4) Nernst, ibid. p. 784.—(i.) and (iv.) Ettinghausen
and Nernst, Wied. Ann. vol. xxix. (1886), p. 343.—(ii.) and (iii.)
Righi, Rend. Acc. Line. vol. iii., part ii. (1887), p. 6, and part i.
P. 481 ; and Leduc, Journ. de Phys. vol. vi. (1887), p. 78.
Additional authorities are quoted by Lloyd, loc. cit.
essentially identical with it, the primary electromotive
force in the case of the latter being that of the Thomson
effect in the unequally heated metal (Ency. Brit. vol. viii.
p. 97), while in the Hall experiment it is derived from an
external source.
Thermo-electric Quality.—According to Kelvin (Thomson)
the thermo-electric quality of iron is affected by longi¬
tudinal magnetization in such a manner that the thermo¬
electric current flows from the unmagnetized to the
magnetized metal through the hot junction; or, in other
words, longitudinal magnetization renders iron less positive
(Ency. Brit. vol. xv. p. 273). It has been found
that when the magnetizing force is increased, this effect
passes a maximum, and in a sufficiently strong field
would probably even be reversed.3 Ewing (Phil. Trans.
vol. clxxvii. p. 373) has shown that tensile stress diminishes
the thermo-electric effect of magnetization and reverses it
when the load is great; nickel always becomes more
positive when magnetized; Tomlinson (Proc. Roy. Soc.
vol. xxxix. (1885), p. 513) found that cobalt in a magnetic
field of 44 units was negative to unmagnetized cobalt.
If it should turn out that the effect upon cobalt is reversed
in a weak field, the interesting fact would be established
that the changes produced by magnetization in the
thermo-electric qualities of the ferromagnetic metals are
exactly parallel to those which it causes in their dimensions.
A comprehensive series of experiments by Lownds (Ann.
d. Phys. vol. iv. (1901), p. 776) shows conclusively, in
opposition to the view long held, that the thermo-electric
quality of bismuth is entirely unaffected by a magnetic
field.
Elasticity.—The results of experiments as to the effect
of magnetization upon elasticity were until quite lately dis¬
cordant and inconclusive, sufficient care not having generally
been taken to protect the specimen under test from the
heat radiated by the magnetizing coil. Recent researches,
however, indicate pretty clearly that for iron and nickel
both Young’s modulus and the rigidity are materially
increased when the metals are subjected to a magnetic
field.4 Day, finding that in very strong fields the rigidity
continued to increase long after magnetic saturation had
been reached, concludes that the phenomena of magnetic
intensity and of magnetic rigidity are quite distinct,
a practical limit to the former being reached in com¬
paratively weak fields. This observation is of interest in
relation to the changes of length produced by magnetism,
though, as Barus remarks, the rigidity, unlike the length,
never passes a maximum. Tangl’s delicate experiments
show that Young’s modulus for a magnetized wire depends
not only upon the magnetizing force, but also upon the
tensile stress. The effects are very complex, and require
further investigation.
Chemical and Voltaic Effects.—If two iron plates, one
of which is magnetized, are immersed in an electrolyte,
a current will generally be indicated by a galvanometer
connected with the plates.
As to whether the magnetized plate becomes positive or negative
to the other, different experimenters are not in agreement. It has,
however, been shown by Hurmuzescn {Rap. du Congres Int. de
Phys., Paris, 1900, p. 561) that the true effect of magnetization is
liable to be disguised by secondary or parasitic phenomena, arising
chiefly from polarization of the electrodes and from local variations
in the concentration and magnetic condition of the electrolyte ;
these may be avoided by working with weak solutions, exposing
only a small surface in a non-polar region of the metal, and sub-
3 Chassagny, C. R. vol. cxvi. (1893), p. 977.—Lala and Fournier,
C. R. vol. cxxiii. (1896), p. 801.—Houlevigne, Journ. de Phys. vol. v.
(1896), p. 53.
4 Day, Am. Journ. Sci. vol. iii. (189/), p. 449. —Stevens and
Dorsay, Pbys. Rev. vol. ix. (1899), p. 116, and vol. x. (1900),
p. 161.—Barus, Am. Journ. Sci. vol. x. (1900), p. 407.—Tangl,
Ann. d. Phys. vol. vi. (1901), p. 34.
449
potential, a current is indicated by the galvanometer.
(See Ency. Brit. vol. xv. p. 273.) The transverse electro¬
motive force is equal to KCH/D, where C is the current,
H the strength of the field, D the thickness of the metal,
and K a constant which has been termed the rotatory
power or rotational coefficient. (See Hopkinson, Phil.
Mag. vol. x. (1880), p. 430.) The following values of
K for different metals are given by Hall (Phil. Mag.
vol. xix. (1885), p. 419), the positive sign indicating that
the electromotive force is in the same direction as the
mechanical force acting upon the conductor. Ettinghausen
Metal.
Antimony
Steel
Iron
Cobalt
Zinc
K x 1015.
+ 114000
+ 12060
+ 7850
+ 2460
+ 820
Metal.
Copper
Gold
Mckel
Bismuth1
K x 1015.
-520
-660
-14740
- 8580000
and Nernst (Wien. Ber. vol. xciv. [2] (1886), p. 560) have
found that the rotational coefficient of tellurium is more
than fifty times greater than that of bismuth, its sign
being positive. Several experimenters have endeavoured
to find a Hall effect in liquids, but such results as have
been hitherto obtained are by no means free from doubt.
Marx (Ann. d. Phys. vol. ii. (1900), p. 798) observed
a well-defined Hall effect in incandescent gases.
Electro-Thermal Relations.—The Hall electromotive
force is only one of several so-called “ galvano-magnetic
effects ” which are observed when a magnetic field acts
normally upon a thin plate of metal traversed by an
electric current. It is remarkable that if a flow of heat
be substituted for a current of electricity, a closely allied
group of “thermo-magnetic effects” is presented. The
two classes of phenomena have been collated by Lloyd
(Am. Journ. Sci. vol. xii. (1901), p. 57) as follows:—
Galvano-Magnetic Effects. Thermo-Magnetic Effects.
(1) A transverse difference of (i.) A transverse difference of
electric potential (Hall effect). electric potential (Nernst effect).
(2) A transverse difference (ii.) A transverse difference
of temperature (Ettinghausen of temperature (Leduc effect),
effect).
(3) Longitudinal change of (iii.) Longitudinal change of
electric conductivity. thermal conductivity.
(4) Longitudinal difference of (iv.) Longitudinal difference
temperature. of electric potential.1 2
If in the annexed diagram ABCD represents the
metallic plate through which the current of electricity
or heat flows in the direction AB, then effects (1), (2),
(i.), and (ii.) are exhibited at C and
D, effects (4) and (iv.) at A and B,
and effects (3) and (iii.) along
AB. The transverse effects are
reversed in direction when either
the magnetic field or the primary current (electric or
thermal) is reversed, but the longitudinal effects are
independent of the direction of the field. Bismuth is
the only metal in which all the phenomena of both
classes have yet been observed. It has been shown by
Moreau (G. R. vol. cxxx. (1900), pp. 122, 412, 562) that
if K is the coefficient of the Hall effect (1) and K' the
analogous coefficient of the Nernst effect (i.) (which is
constant for small values of H), then K' = Kcr/p, a being
the coefficient of the Thomson effect for the metal and
p its specific resistance. He considers that Hall’s is the
fundamental phenomenon, and that the Nernst effect is
C
A B :
D
1 The large Hall effect in bismuth was discovered by Righi {Journ.
de Phys. [2], vol. iii. (1884), p. 127).
2 References.—(2) Ettinghausen, Wied. Ann. vol. xxxi. (1887),
p. 737.—(4) Nernst, ibid. p. 784.—(i.) and (iv.) Ettinghausen
and Nernst, Wied. Ann. vol. xxix. (1886), p. 343.—(ii.) and (iii.)
Righi, Rend. Acc. Line. vol. iii., part ii. (1887), p. 6, and part i.
P. 481 ; and Leduc, Journ. de Phys. vol. vi. (1887), p. 78.
Additional authorities are quoted by Lloyd, loc. cit.
essentially identical with it, the primary electromotive
force in the case of the latter being that of the Thomson
effect in the unequally heated metal (Ency. Brit. vol. viii.
p. 97), while in the Hall experiment it is derived from an
external source.
Thermo-electric Quality.—According to Kelvin (Thomson)
the thermo-electric quality of iron is affected by longi¬
tudinal magnetization in such a manner that the thermo¬
electric current flows from the unmagnetized to the
magnetized metal through the hot junction; or, in other
words, longitudinal magnetization renders iron less positive
(Ency. Brit. vol. xv. p. 273). It has been found
that when the magnetizing force is increased, this effect
passes a maximum, and in a sufficiently strong field
would probably even be reversed.3 Ewing (Phil. Trans.
vol. clxxvii. p. 373) has shown that tensile stress diminishes
the thermo-electric effect of magnetization and reverses it
when the load is great; nickel always becomes more
positive when magnetized; Tomlinson (Proc. Roy. Soc.
vol. xxxix. (1885), p. 513) found that cobalt in a magnetic
field of 44 units was negative to unmagnetized cobalt.
If it should turn out that the effect upon cobalt is reversed
in a weak field, the interesting fact would be established
that the changes produced by magnetization in the
thermo-electric qualities of the ferromagnetic metals are
exactly parallel to those which it causes in their dimensions.
A comprehensive series of experiments by Lownds (Ann.
d. Phys. vol. iv. (1901), p. 776) shows conclusively, in
opposition to the view long held, that the thermo-electric
quality of bismuth is entirely unaffected by a magnetic
field.
Elasticity.—The results of experiments as to the effect
of magnetization upon elasticity were until quite lately dis¬
cordant and inconclusive, sufficient care not having generally
been taken to protect the specimen under test from the
heat radiated by the magnetizing coil. Recent researches,
however, indicate pretty clearly that for iron and nickel
both Young’s modulus and the rigidity are materially
increased when the metals are subjected to a magnetic
field.4 Day, finding that in very strong fields the rigidity
continued to increase long after magnetic saturation had
been reached, concludes that the phenomena of magnetic
intensity and of magnetic rigidity are quite distinct,
a practical limit to the former being reached in com¬
paratively weak fields. This observation is of interest in
relation to the changes of length produced by magnetism,
though, as Barus remarks, the rigidity, unlike the length,
never passes a maximum. Tangl’s delicate experiments
show that Young’s modulus for a magnetized wire depends
not only upon the magnetizing force, but also upon the
tensile stress. The effects are very complex, and require
further investigation.
Chemical and Voltaic Effects.—If two iron plates, one
of which is magnetized, are immersed in an electrolyte,
a current will generally be indicated by a galvanometer
connected with the plates.
As to whether the magnetized plate becomes positive or negative
to the other, different experimenters are not in agreement. It has,
however, been shown by Hurmuzescn {Rap. du Congres Int. de
Phys., Paris, 1900, p. 561) that the true effect of magnetization is
liable to be disguised by secondary or parasitic phenomena, arising
chiefly from polarization of the electrodes and from local variations
in the concentration and magnetic condition of the electrolyte ;
these may be avoided by working with weak solutions, exposing
only a small surface in a non-polar region of the metal, and sub-
3 Chassagny, C. R. vol. cxvi. (1893), p. 977.—Lala and Fournier,
C. R. vol. cxxiii. (1896), p. 801.—Houlevigne, Journ. de Phys. vol. v.
(1896), p. 53.
4 Day, Am. Journ. Sci. vol. iii. (189/), p. 449. —Stevens and
Dorsay, Pbys. Rev. vol. ix. (1899), p. 116, and vol. x. (1900),
p. 161.—Barus, Am. Journ. Sci. vol. x. (1900), p. 407.—Tangl,
Ann. d. Phys. vol. vi. (1901), p. 34.
Set display mode to:
Universal Viewer |
Mirador |
Large image | Transcription
Images and transcriptions on this page, including medium image downloads, may be used under the Creative Commons Attribution 4.0 International Licence unless otherwise stated.
Encyclopaedia Britannica > New volumes of the Encyclopædia Britannica > Volume 30, K-MOR > (479) Page 449 |
---|
Permanent URL | https://digital.nls.uk/193573728 |
---|
Attribution and copyright: |
|
---|---|
![]() |
Shelfmark | EB.18 |
---|---|
![]() |
Description | Ten editions of 'Encyclopaedia Britannica', issued from 1768-1903, in 231 volumes. Originally issued in 100 weekly parts (3 volumes) between 1768 and 1771 by publishers: Colin Macfarquhar and Andrew Bell (Edinburgh); editor: William Smellie: engraver: Andrew Bell. Expanded editions in the 19th century featured more volumes and contributions from leading experts in their fields. Managed and published in Edinburgh up to the 9th edition (25 volumes, from 1875-1889); the 10th edition (1902-1903) re-issued the 9th edition, with 11 supplementary volumes. |
---|---|
Additional NLS resources: |
|