New volumes of the Encyclopædia Britannica > Volume 30, K-MOR
(49) Page 27 - KEL
Download files
Complete book:
Individual page:
Thumbnail gallery: Grid view | List view
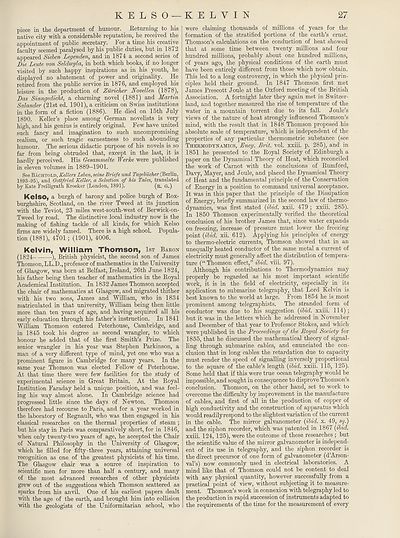
KELSO —
piece in the department of humour. Returning to his
native city with a considerable reputation, he received the
appointment of public secretary. For a time his creative
faculty seemed paralysed by his public duties, but in. 1872
appeared Sieben Lagenden, and in 1874 a second series of
Die Leute von Seldwyla, in both which books,_ if no longer
visited by such happy inspirations as in his youth, he
displayed no abatement of power and originality. He
retired from the public service in 1876, and employed his
leisure in the production of Zuricher Novellen (1878),
Das Sinngedicht, a charming novel (1881) and Martin
Salander (21st ed. 1901), a criticism on Swiss institutions
in the form of a fiction (1886). He died on 15th July
1890. Keller’s place among German novelists is very
high, and his genius is entirely original. Few have united
such fancy and imagination to such uncompromising
realism, or such tragic earnestness to such abounding
humour. The serious didactic purpose of his novels is so
far from being obtruded that, except in the last, it is
hardly perceived. His Gesammelte Werke were published
in eleven volumes in 1889-1901.
See Bachtold, Kellers Leben, seine Briefe und Tagebucher (Berlin,
1893-95), and Gottfried Keller, a Selection of his Tales, translated
by Kate Freiligrath Kroeker (London, 1891). (k. g.)
KelSO, a burgh of barony and police burgh of Rox¬
burghshire, Scotland, on the river Tweed at its junction
with the Teviot, 23 miles west-south-west of Berwick-on-
Tweed by road. The distinctive local industry now is the
making of fishing tackle of all kinds, for which Kelso
firms are widely famed. There is a high school. Popula¬
tion (1881), 4701 ; (1901), 4006.
Kelvin, William Thomson, 1st Baron
(1824 ), British physicist, the second son of James
Thomson, LL.D., professor of mathematics in the University
of Glasgow, was born at Belfast, Ireland, 26th June 1824,
his father being then teacher of mathematics in the Royal
Academical Institution. In 1832 James Thomson accepted
the chair of mathematics at Glasgow, and migrated thither
with his two sons, James and William, who in 1834
matriculated in that university, William being then little
more than ten years of age, and having acquired all his
early education through his father’s instruction. In 1841
William Thomson entered Peterhouse, Cambridge, and
in 1845 took his degree as second wrangler, to which
honour he added that of the first Smith’s Prize. The
senior wrangler in his year was Stephen Parkinson, a
man of a very different type of mind, yet one who was a
prominent figure in Cambridge for many years. In the
same year Thomson was elected Fellow of Peterhouse.
At that time there were few facilities for the study of
experimental science in Great Britain. At the Royal
Institution Faraday held a unique position, and was feel¬
ing his way almost alone. In Cambridge science had
progressed little since the days of Newton. Thomson
therefore had recourse to Paris, and for a year worked in
the laboratory of Regnault, who was then engaged in his
classical researches on the thermal properties of steam ;
but his stay in Paris was comparatively short, for in 1846,
when only twenty-two years of age, he accepted the Chair
of Natural Philosophy in the University of Glasgow,
which he filled for fifty-three years, attaining universal
recognition as one of the greatest physicists of his time.
The Glasgow chair was a source of inspiration to
scientific men for more than half a century, and many
of the most advanced researches of other physicists
grew out of the suggestions which Thomson scattered as
sparks from his anvil. One of his earliest papers dealt
with the age of the earth, and brought him into collision
Avith the geologists of the Uniformitarian school, who
KELVIN 27
were claiming thousands of millions of years for the
formation of the stratified portions of the earth’s crust.
Thomson’s calculations on the conduction of heat showed
that at some time between twenty millions and four
hundred millions, probably about one hundred millions,
of years ago, the physical conditions of the earth must
have been entirely different from those which now obtain.
This led to a long controversy, in which the physical prin¬
ciples held their ground. In 1847 Thomson first met
James Prescott Joule at the Oxford meeting of the British
Association. A fortnight later they again met in Switzer¬
land, and together measured the rise of temperature of the
water in a mountain torrent due to its fall. Joule’s
views of the nature of heat strongly influenced Thomson’s
mind, with the result that in 1848 Thomson proposed his
absolute scale of temperature, which is independent of the
properties of any particular thermometric substance (see
Thermodynamics, Ency. Brit. vol. xxiii. p. 285), and in
1851 he presented to the Royal Society of Edinburgh a
paper on the Dynamical Theory of Heat, which reconciled
the work of Carnot with the conclusions of Rumford,
Da\y, Mayer, and Joule, and placed the Dynamical Theory
of Heat and the fundamental principle of the Conservation
of Energy in a position to command universal acceptance.
It was in this paper that the principle of the Dissipation
of Energy, briefly summarized in the second law of thermo¬
dynamics, was first stated {ibid. xxii. 479 ; xxiii. 285).
In 1850 Thomson experimentally verified the theoretical
conclusion of his brother James that, since water expands
on freezing, increase of pressure must lower the freezing
point {ibid. xii. 612). Applying his principles of energy
to thermo-electric currents, Thomson showed that in an
unequally heated conductor of the same metal a current of
electricity must generally affect the distribution of tempera¬
ture (“ Thomson effect,” ibid. viii. 97).
Although his contributions to Thermodynamics may
properly be regarded as his most important scientific
work, it is in the field of electricity, especially in its
application to submarine telegraphy, that Lord Kelvin is
best known to the world at large. From 1854 he is most
prominent among telegraphists. The stranded form of
conductor was due to his suggestion {ibid, xxiii. 114);
but it was in the letters which he addressed in November
and December of that year to Professor Stokes, and which
were published in the Proceedings of the Royal Society for
1855, that he discussed the mathematical theory of signal¬
ling through submarine cables, and enunciated the con¬
clusion that in long cables the retardation due to capacity
must render the speed of signalling inversely proportional
to the square of the cable’s length {ibid, xxiii. 115, 125).
Some held that if this were true ocean telegraphy would be
impossible, and sought in consequence to disprove Thomson’s
conclusion. Thomson, on the other hand, set to work to
overcome the difficulty by improvement in the manufacture
of cables, and first of all in the production of copper of
high conductivity and the construction of apparatus which
would readily respond to the slightest variation of the current
in the cable. The mirror galvanometer {ibid. x. 49, sg.)
and the siphon recorder, which was patented in 1867 {ibid.
xxiii. 124, 125), were the outcome of these researches; but
the scientific value of the mirror galvanometer is independ¬
ent of its use in telegraphy, and the siphon recorder is
the direct precursor of one form of galvanometer (d’Arson-
val’s) now commonly used in electrical laboratories. A
mind like that of Thomson could not be content to deal
with any physical quantity, however successfully from a
practical point of view, without subjecting it to measure¬
ment. Thomson’s work in connexion with telegraphy led to
the production in rapid succession of instruments adapted to
the requirements of the time for the measurement of every
piece in the department of humour. Returning to his
native city with a considerable reputation, he received the
appointment of public secretary. For a time his creative
faculty seemed paralysed by his public duties, but in. 1872
appeared Sieben Lagenden, and in 1874 a second series of
Die Leute von Seldwyla, in both which books,_ if no longer
visited by such happy inspirations as in his youth, he
displayed no abatement of power and originality. He
retired from the public service in 1876, and employed his
leisure in the production of Zuricher Novellen (1878),
Das Sinngedicht, a charming novel (1881) and Martin
Salander (21st ed. 1901), a criticism on Swiss institutions
in the form of a fiction (1886). He died on 15th July
1890. Keller’s place among German novelists is very
high, and his genius is entirely original. Few have united
such fancy and imagination to such uncompromising
realism, or such tragic earnestness to such abounding
humour. The serious didactic purpose of his novels is so
far from being obtruded that, except in the last, it is
hardly perceived. His Gesammelte Werke were published
in eleven volumes in 1889-1901.
See Bachtold, Kellers Leben, seine Briefe und Tagebucher (Berlin,
1893-95), and Gottfried Keller, a Selection of his Tales, translated
by Kate Freiligrath Kroeker (London, 1891). (k. g.)
KelSO, a burgh of barony and police burgh of Rox¬
burghshire, Scotland, on the river Tweed at its junction
with the Teviot, 23 miles west-south-west of Berwick-on-
Tweed by road. The distinctive local industry now is the
making of fishing tackle of all kinds, for which Kelso
firms are widely famed. There is a high school. Popula¬
tion (1881), 4701 ; (1901), 4006.
Kelvin, William Thomson, 1st Baron
(1824 ), British physicist, the second son of James
Thomson, LL.D., professor of mathematics in the University
of Glasgow, was born at Belfast, Ireland, 26th June 1824,
his father being then teacher of mathematics in the Royal
Academical Institution. In 1832 James Thomson accepted
the chair of mathematics at Glasgow, and migrated thither
with his two sons, James and William, who in 1834
matriculated in that university, William being then little
more than ten years of age, and having acquired all his
early education through his father’s instruction. In 1841
William Thomson entered Peterhouse, Cambridge, and
in 1845 took his degree as second wrangler, to which
honour he added that of the first Smith’s Prize. The
senior wrangler in his year was Stephen Parkinson, a
man of a very different type of mind, yet one who was a
prominent figure in Cambridge for many years. In the
same year Thomson was elected Fellow of Peterhouse.
At that time there were few facilities for the study of
experimental science in Great Britain. At the Royal
Institution Faraday held a unique position, and was feel¬
ing his way almost alone. In Cambridge science had
progressed little since the days of Newton. Thomson
therefore had recourse to Paris, and for a year worked in
the laboratory of Regnault, who was then engaged in his
classical researches on the thermal properties of steam ;
but his stay in Paris was comparatively short, for in 1846,
when only twenty-two years of age, he accepted the Chair
of Natural Philosophy in the University of Glasgow,
which he filled for fifty-three years, attaining universal
recognition as one of the greatest physicists of his time.
The Glasgow chair was a source of inspiration to
scientific men for more than half a century, and many
of the most advanced researches of other physicists
grew out of the suggestions which Thomson scattered as
sparks from his anvil. One of his earliest papers dealt
with the age of the earth, and brought him into collision
Avith the geologists of the Uniformitarian school, who
KELVIN 27
were claiming thousands of millions of years for the
formation of the stratified portions of the earth’s crust.
Thomson’s calculations on the conduction of heat showed
that at some time between twenty millions and four
hundred millions, probably about one hundred millions,
of years ago, the physical conditions of the earth must
have been entirely different from those which now obtain.
This led to a long controversy, in which the physical prin¬
ciples held their ground. In 1847 Thomson first met
James Prescott Joule at the Oxford meeting of the British
Association. A fortnight later they again met in Switzer¬
land, and together measured the rise of temperature of the
water in a mountain torrent due to its fall. Joule’s
views of the nature of heat strongly influenced Thomson’s
mind, with the result that in 1848 Thomson proposed his
absolute scale of temperature, which is independent of the
properties of any particular thermometric substance (see
Thermodynamics, Ency. Brit. vol. xxiii. p. 285), and in
1851 he presented to the Royal Society of Edinburgh a
paper on the Dynamical Theory of Heat, which reconciled
the work of Carnot with the conclusions of Rumford,
Da\y, Mayer, and Joule, and placed the Dynamical Theory
of Heat and the fundamental principle of the Conservation
of Energy in a position to command universal acceptance.
It was in this paper that the principle of the Dissipation
of Energy, briefly summarized in the second law of thermo¬
dynamics, was first stated {ibid. xxii. 479 ; xxiii. 285).
In 1850 Thomson experimentally verified the theoretical
conclusion of his brother James that, since water expands
on freezing, increase of pressure must lower the freezing
point {ibid. xii. 612). Applying his principles of energy
to thermo-electric currents, Thomson showed that in an
unequally heated conductor of the same metal a current of
electricity must generally affect the distribution of tempera¬
ture (“ Thomson effect,” ibid. viii. 97).
Although his contributions to Thermodynamics may
properly be regarded as his most important scientific
work, it is in the field of electricity, especially in its
application to submarine telegraphy, that Lord Kelvin is
best known to the world at large. From 1854 he is most
prominent among telegraphists. The stranded form of
conductor was due to his suggestion {ibid, xxiii. 114);
but it was in the letters which he addressed in November
and December of that year to Professor Stokes, and which
were published in the Proceedings of the Royal Society for
1855, that he discussed the mathematical theory of signal¬
ling through submarine cables, and enunciated the con¬
clusion that in long cables the retardation due to capacity
must render the speed of signalling inversely proportional
to the square of the cable’s length {ibid, xxiii. 115, 125).
Some held that if this were true ocean telegraphy would be
impossible, and sought in consequence to disprove Thomson’s
conclusion. Thomson, on the other hand, set to work to
overcome the difficulty by improvement in the manufacture
of cables, and first of all in the production of copper of
high conductivity and the construction of apparatus which
would readily respond to the slightest variation of the current
in the cable. The mirror galvanometer {ibid. x. 49, sg.)
and the siphon recorder, which was patented in 1867 {ibid.
xxiii. 124, 125), were the outcome of these researches; but
the scientific value of the mirror galvanometer is independ¬
ent of its use in telegraphy, and the siphon recorder is
the direct precursor of one form of galvanometer (d’Arson-
val’s) now commonly used in electrical laboratories. A
mind like that of Thomson could not be content to deal
with any physical quantity, however successfully from a
practical point of view, without subjecting it to measure¬
ment. Thomson’s work in connexion with telegraphy led to
the production in rapid succession of instruments adapted to
the requirements of the time for the measurement of every
Set display mode to:
Universal Viewer |
Mirador |
Large image | Transcription
Images and transcriptions on this page, including medium image downloads, may be used under the Creative Commons Attribution 4.0 International Licence unless otherwise stated.
Encyclopaedia Britannica > New volumes of the Encyclopædia Britannica > Volume 30, K-MOR > (49) Page 27 - KEL |
---|
Permanent URL | https://digital.nls.uk/193568138 |
---|
Attribution and copyright: |
|
---|---|
![]() |
Shelfmark | EB.18 |
---|---|
![]() |
Description | Ten editions of 'Encyclopaedia Britannica', issued from 1768-1903, in 231 volumes. Originally issued in 100 weekly parts (3 volumes) between 1768 and 1771 by publishers: Colin Macfarquhar and Andrew Bell (Edinburgh); editor: William Smellie: engraver: Andrew Bell. Expanded editions in the 19th century featured more volumes and contributions from leading experts in their fields. Managed and published in Edinburgh up to the 9th edition (25 volumes, from 1875-1889); the 10th edition (1902-1903) re-issued the 9th edition, with 11 supplementary volumes. |
---|---|
Additional NLS resources: |
|